Introduction¶
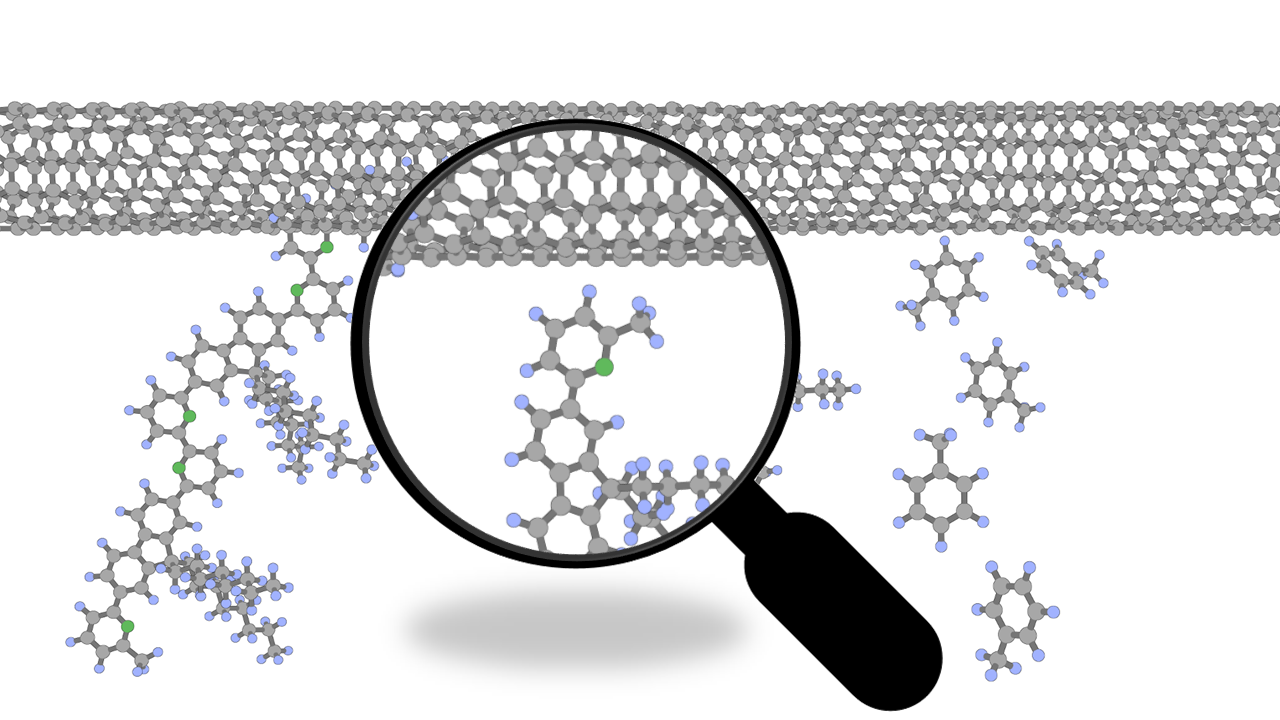
Molecular Adsorptions on a Single-Wall Carbon Nanotube.¶
Down-scaling of electronic circuits has undoubtedly determined the evolution of technology in the past 50 years. For decades, the semiconductor industry relied on an exponential growth predicted by Moore in 1965 [1]. While being a landmark for the prediction of demands in information technology, Moore’s Law is at its limit in current silicon technology. Economic pressure has forced mass production in 7nm technology stacking circuits in three dimensions, but further down-scaling of the two-dimensional base circuits is not likely to happen in silicon-based fabrication technology. Bringing the production of electronics below the 10nm limit will require the use of new technologies for electronic components.
Carbon nanotubes have attracted considerable attention due to their defined nano-scaled structure and strongly varying electronic properties. A long search for synthesis conditions finally led to an excellent control over the process using sustainable carbon sources.[2] The electronic properties originate from monolayer graphene and are highly adjustable to give either semiconducting or metallic nanotubes. The diameter and roll-up angle of a nanotube control the band structure by changing the symmetric arrangement of p-orbitals. As the diameter gets smaller, electronic effects become more prominent, and changes in the dielectric medium have a higher impact.[3]
Recent improvements to the synthesis [4][5] allow much better control of the production process and significantly increase the number of nanotubes with diameters and emission wavelengths that are below those of the (6,5) type. Today, almost every synthesis of semiconducting carbon nanotubes leads to a mixture of nanotubes with similar diameters. For applications like the production of transistors, it is, however, favorable to produce only specific types. Preselection can be performed through synthesis, but today, such selection is better achieved through wet processing with polymers [6] that selectively adsorb on the surface of specific types and remove metallic nanotubes. This adsorption is a combination of solvent, π-stacking, and dispersion forces and is not fully understood.[7] A more detailed look into these adsorption mechanisms could give a better insight for more efficient separation of nanotube types.
Nanotube-based electronics are an alternative to silicon-based electronics. Such electronics are down-scalable, do not require the use of rare earth metals to achieve their unique properties, and can be synthesized from sustainable precursors. Metallic nanotubes can be used to transport charges and semiconducting nanotubes to form electronic components. In 2012, IBM presented the first implementation of semiconducting carbon nanotubes as a transistor in a sub-10nm geometry[8]. These investigations enabled to build the first operable computer [9] using carbon nanotubes as transistors. To increase computation power, a caching memory for storing intermediate calculation results, and a power source are crucial. Supercapacitors fulfill both of these needs. Following theoretical predictions, carbon nanotubes are very suitable as electrodes in supercapacitors [10]. Research on all-carbon electronics should, therefore, be highly favorable. However, almost no experimental evidence for the usage of carbon nanotubes as supercapacitors are available since adsorption processes on the single nanotube level are not so easily be observed and are generally hard to understand.
The photoluminescence of semiconducting carbon nanotubes is in the short wave infrared (SWIR) region. This region is important for enhancing the image contrast in biological samples as it avoids blood and water absorptions typically present in biological tissue.[11] Also, glass fibers do not show absorption in the SWIR range, which enables a better transmission of data at telecom wavelengths. Moreover, the photoluminescence has shown single-photon emission [12][13], which enables the use of semiconducting carbon nanotubes in quantum cryptography. Such a transmission technique is operable on any existing fiber glass network. As carbon nanotubes also show electroluminescence with high carrier-to-photon conversion efficiencies [14] nano-scaled lasing components became possible that can be coupled efficiently to wave-guides. It was also recently found that sp³-defects highly enhance these emission properties. These defects both show luminescence up-conversion in biological tissues [15], and photon anti-bunching [16][17][18] at room temperature. Such defects can be efficiently synthesized through chemisorbed hydrogen [19] on the surface of nanotubes.
The highest culprit, however, is the generally low photoluminescence quantum yield of nanotubes that is caused by further irradiative decay paths in these systems. The transfer to solvent for wet processing, for example, leads to a major loss in nanotube emission through non-radiative decay paths of the excitonic states. The structural arrangement of such molecules on a carbon nanotube can also be used to form various properties. The emission strength of these excitons is highly influenced by physisorbed molecules on the nanotube surface.
Carbon Nanotubes only consist of surface atoms and exhibit a high surface to volume ratio. All nanotube-based technology is, therefore, based on surface adsorptions. In the following, I will investigate these surface interactions on the single-molecule level focussing on nanotubes with diameters of 0.7-1.2nm. It will be shown that the initial gas adsorption that is inherent in air significantly shifts the emission energy. Gas adsorption is also responsible for the introduction of new states through sp³-defects. Further findings allow us to estimate the quantum yield loss in different solvents and help to shed light on the different processes during polymer adsorption that are responsible for a selective sorting of specific carbon nanotube roll-up angles. The first chapter focusses on the experiment design for the synthesis and microscopic investigation of suspended nanotubes which allows making statistically significant analyses. It starts by giving a short introduction on the history of nanotube synthesis before introducing the setup.